Advertisement
9. The Fascinating Fractal Nature of Foam: From Lattes to Ocean Waves
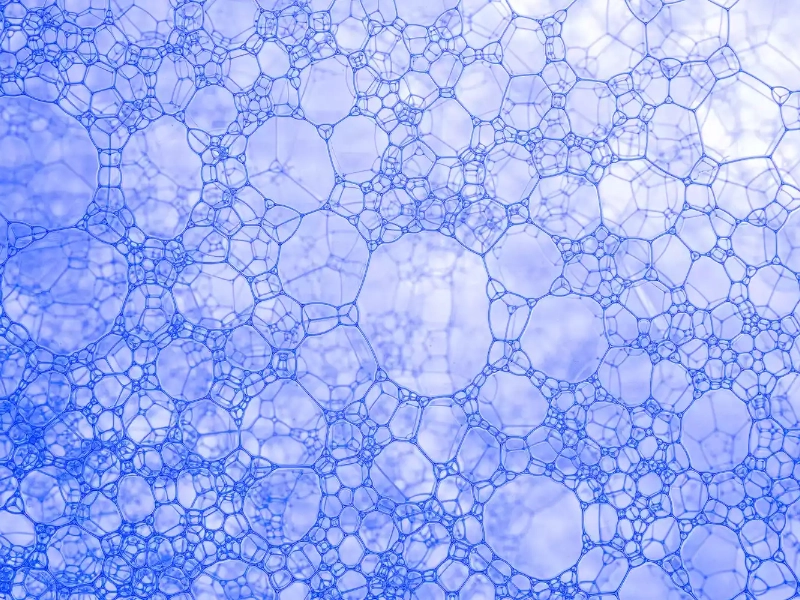
Advertisement
Though we come with this apparently basic material in many different shapes in our daily life, foam has a complicated and fascinating fractal structure that has enthralled mathematicians and physicists equally. From the frothy top of your morning cappuccino to the white caps of ocean waves, foam shows an amazing self-similarity across many sizes—a feature unique of fractals. Not only a visual wonder, this fractal character is a basic feature of foam's structure and behaviour with consequences ranging from the microscopic to the macroscopic level. Fundamentally, foam is a unique substance combining characteristics of both phases by means of a dispersion of gas bubbles in a liquid or solid media. Surface tension, gravity, and bubble formation and coalescence interact to create the fractal structure of foam. Larger bubbles surrounded by ever smaller ones, each level reflecting the structure of the whole, generate a hierarchy of sizes as bubbles interact. This self-similar pattern recurs over several orders of magnitude in size. The outcome is a delicate and remarkably stable labyrinthine structure formed by a complicated network of thin liquid films separating gas pockets of varied size. Foam's fractal character is dynamic rather than only static; it is always changing as bubbles combine, split, and reorganise in response to both internal and external stresses. This dynamic behaviour adds to the remarkable qualities of foam, such its capacity to flow like a liquid while preserving a structure more like a solid.
Physical and chemical characteristics of foam depend much on its fractal structure. For example, the hierarchical organisation of bubbles traps air at several levels and reduces heat transfer, hence contributing to the extraordinary insulating power of foam. From the foam insulation in our homes to the frothy head on a beer that helps retain its temperature and aroma, this quality finds use in many different contexts. Furthermore affecting its mechanical characteristics is foam's fractal character, which enables it to absorb energy and distort under stress in different ways than liquids and solids. For uses ranging from protective packaging to shock-absorbing sports equipment, foam's unusual behaviour makes it the perfect material. Finding uses in everything from firefighting to oil recovery to wastewater treatment, foam is a great medium for chemical reactions and mass transfer processes thanks in great part to the large surface area generated by the fractal network of bubble interfaces. Where comparable patterns are seen in the large-scale structure of the universe, research of foam's fractal structure has advanced materials science, fluid dynamics, and even cosmology.
Nature is full of foam, a widespread phenomena with unique fractal patterns that define this interesting material in many different settings. One of the most obvious and dynamic forms of natural foam are ocean waves breaking on a coast. Waves entrain air as they crest and crash, creating a complicated network of bubbles spanning both minute inclusions and big, obvious pockets. Through its impact on albedo, the reflectance of the Earth's surface, this foam is essential for the ocean ecology since it influences gas exchange between the atmosphere and the water, so affecting microbiological distribution, and even global climate. Sea foam's fractal structure maximises surface area for interactions and generates a gradient of bubble sizes capable of trapping and moving different chemicals, therefore augmenting these effects. In the same manner, raindrops on bodies of water or moist soil produce small foam structures displaying the same self-similar patterns seen in more massive foam formations. Though brief, these transient foam structures can have major effects on nearby surroundings, hence affecting the aeration of water bodies and nutrient distribution in soil. The fractal character of this rain-induced foam improves its capacity to absorb and disseminate particles and gases, therefore supporting processes ranging from soil erosion to the dissemination of waterborne diseases.
Foam structures are found in many contexts in biological systems, usually fulfilling specialised purposes using the particular qualities of fractal geometries. For instance, the foam nests made by some frog species exemplify how nature has developed to take use of fractal foam structures' insulating and protecting qualities. Comprising proteins and other biological substances whipped into a stable foam by the frogs, these nests offer a regulated microenvironment for egg development, predator protection, desiccation avoidance, and temperature control. These nests' fractal structure of bubbles maximises their insulating qualities and allows gas exchange, therefore proving the effectiveness of natural design. In plant biology, the aerenchyma tissue seen in aquatic plants shows a foam-like structure that helps gas exchange between submerged sections of the plant and the atmosphere. The fractal arrangement of this tissue maximises surface area for gas transport and reduces structural material needed, therefore enabling plants to flourish in wet conditions. Even in the field of microbiology, some bacterial colonies create biofilms with foam-like structures that show fractal characteristics, therefore improving their durability and capacity to interact with their surroundings for waste product and nutritional exchange.
Beyond its natural occurrences, foam is a ubiquitous presence in our daily life and shows itself in both very specialised and ordinary settings. For many individuals, the most known artificial foam is probably the soap bubbles created while washing dishes or bathing. Actually, these apparently basic constructions are sophisticated systems displaying surface chemistry and fractal geometry. Agitating soapy water produces a hierarchy of bubbles with self-similar arrangement whereby bigger bubbles are surrounded by ever smaller ones. This fractal form not only looks good but also has useful functions. Dish soap foam's several layers of bubbles, for example, capture dirt particles and oils inside the complex network of liquid films, therefore improving its cleaning power. This foam's fractal character also helps to explain its stability, which lets it keep its structure long enough to be useful for cleaning chores. The fractal structure of foam is precisely created in personal care products such shaving cream and hair mousse to offer particular qualities including spreadability, adhesion to surfaces, and controlled release of active chemicals.
More complex applications make use of foam's fractal qualities for a variety of technical and industrial uses. For instance, especially designed foams are used in firefighting to battle different kinds of flames. By using their fractal structure, these foams simultaneously cool the fire and stop oxygen from getting to the fuel source while forming a stable blanket over burning surfaces. These foams' hierarchical bubble organisation lets them flow and spread efficiently while also preserving their integrity under very demanding conditions. Metal foams with fractal structures are being developed in materials science for uses ranging from lightweight structural components to highly performing heat exchangers. By using their fractal foam structure to take advantage of low density and great surface area, these materials accomplish qualities either difficult or unachievable with conventional solid materials. Chefs and food scientists in the fields of food science and culinary arts are investigating how fractal foams might produce unique textures and taste sensations. Creating stable foams with intricate fractal patterns that can improve the sensory qualities of food and yet allow for creative presentations is a common component of molecular gastronomy methods.
Modern disciplines including nanotechnology and healthcare find application and study in artificial foams with fractal characteristics. For use in enhanced catalysis, energy storage, and drug delivery systems, researchers are creating nanofoams with exactly regulated fractal shapes. Using the great surface area and hierarchical pore structure of fractal foams, these materials attain hitherto unheard-of degrees of performance in their particular uses. Scaffolds with foam-like fractal structures are under investigation in tissue engineering as a way to produce synthetic tissues and organs more faithfully replicas of the intricate architectures seen in genuine biological systems. These scaffolds' fractal character lets nutrients be distributed optimally and cell development possible, so transforming organ transplantation and regenerative medicine. Deepening knowledge of the fractal characteristics of foam will lead to even more creative uses that challenge the limits of what is feasible in disciplines ranging from environmental restoration to space exploration.